advancing the mitigation of Climate Change and Global Warming through Geoengineering education and research
What is Blue Carbon?
Blue Carbon is a natural climate solution and climate engineering approach to mitigate and reverse the effects of Climate Change and Global Warming. Blue Carbon Ecosystems are coastal, estuarine and marine photosynthetic ecosystems that have the capability to absorb significant amounts of carbon dioxide from the atmosphere or environment and store it for long periods of time (i.e., carbon sequestration or biosequestration). Blue Carbon Ecosystems include saltmarshes, seagrass meadows, mangrove forests, macroalgae communities such as kelp forests, and freshwater tidal ecosystems such as coastal bald cypress floodplain forests (Lovelock and Duarte, 2019). Coral and oyster reefs, and some red algae (macroalgae) are not currently considered blue carbon ecosystems because these communities produce calcium carbonate or limestone. The process of creating calcium carbonate (calcification) produces carbon dioxide which could potentially increase the amount of carbon dioxide in the atmosphere.
How do Blue Carbon Ecosystems sequester or store carbon?
Blue Carbon Ecosystems are very important for carbon sequestration to mitigate Climate Change because they are extremely productive and can store carbon for thousands of years. Seagrass meadows, mangrove forests and salt marshes, which cover approximately 0.5% of the area of the world’s oceans, account for more than 50% of all blue carbon and carbon sequestration in ocean sediments (Nellemann et al., 2010). In these blue carbon systems, numerous grass blades of seagrass meadows and salt marshes and prop roots in mangrove forests slow down water currents and capture particles that drop or fall out of these slowed currents. Overtime, this process causes the buildup of the soils and the burying of carbon-containing organic materials (dead plant material, etc.). Because these soils are submerged, they are anaerobic (without oxygen) and are not subjected to periodic fires like terrestrial ecosystems. This results in very little decomposition (or loss of carbon to the atmosphere by burning) and the buildup and long-term sequestration of blue carbon. Seagrass meadows, mangrove forests and salt marshes build soil at a median rate of 5.5, 4.5 and 1.48 millimeters per year, respectively (Duarte et al., 2013/Alongi, 2012).
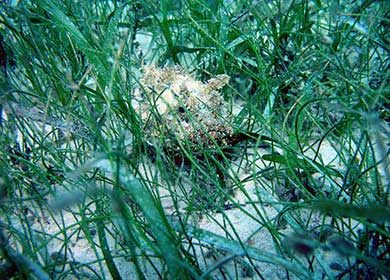
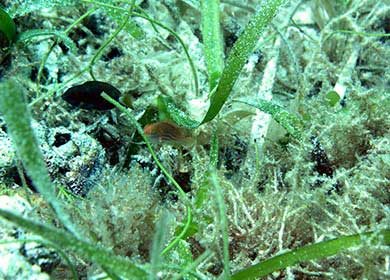
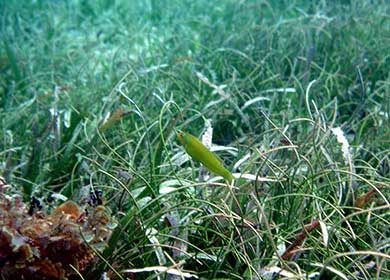
Seagrass Meadows
Seagrass meadows are blue carbon ecosystems that cover between 177,000 to 600,000 km² world-wide (or about 0.2%) of most of the world’s oceans and seas (Green and Short, 2003). Seagrass meadows consist of one or more seagrass species as well as other primary producers including macroalgae and epiphytic algae (algae that grow on the surface of seagrasses or other objects). Seagrass meadows are critically important food sources for dugongs, manatees, sea turtles and waterfowl. These blue carbon ecosystems also support other marine species including grouper, snapper, flounder, sea horses, crabs, horseshoe crabs, lobster, shrimp, scallops, oysters, conchs, mussels, clams, starfish, brittle stars and sea cucumbers (Green and Short, 2003).
How much Blue Carbon do Seagrass Meadows store in their leaves and soil?
Seagrass meadows generally sequester significant amounts of blue carbon in the top meter of soil and, in the Mediterranean Sea, their soils are up to 11 meters thick (Lo Iacono et al., 2008). Seagrass meadows sequester blue carbon by storing carbon in living seagrass leaves (2.52 Mg C per hectare or about 1/3 of the blue carbon) and storing the other 2/3 (329.5 Mg C per hectare) in the soil as live and dead roots and buried organic matter (seagrass leaves, algae and organic material transported from other marine communities) (Fourqurean et al., 2012). Seagrass meadow soils store about twice the average carbon per hectare as terrestrial soils (Fourqurean et al., 2012).
Globally, seagrass meadows currently sequester 75.5 to 151 Tg of carbon in seagrass biomass and up to 19.9 Pg of carbon in seagrass soils. 19.9 Pg of sequestered blue carbon is roughly equal to what is stored globally in tidal marshes and mangrove forests combined (Fourqurean et al., 2012).
What are the threats to Seagrass Meadows?
Seagrass meadows have been disappearing at an average rate of 1.5% per year since the beginning of the 20th Century. This 29% loss has been primarily caused by the degradation of water quality (e.g., coastal eutrophication (nutrient runoff) and reduced light penetration) resulting from poor land-use practices such as deforestation (Duarte, 2002/Short and Wyllie-Echeverria, 1996). The destruction of seagrass meadows has led to the decrease in carbon sequestration by 6 to 24 Tg C per year (Duarte et al., 2010). In the near future, this loss could lead to greenhouse gas emissions rather than carbon sequestration and the release of up to 299 Tg of blue carbon per year (Short and Wyllie-Echeverria, 1996).
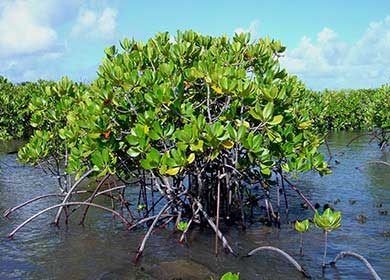
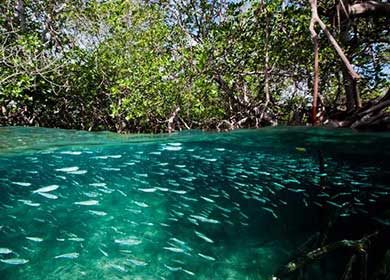
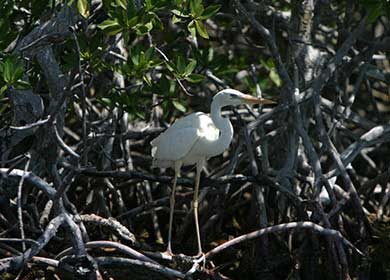
Mangrove Forests
Mangrove forests (see Smithsonian Institute website) are blue carbon ecosystems that located at the interface of the land and sea in the coastal intertidal zone of subtropical and tropical areas of the world. They cover approximately 137,760 to 152,000 km² world-wide (Giri et al., 2011/Donato et al., 2011). Mangrove forests consist of about 70 species of trees, shrubs and ferns that are adapted to grow in shallow seawater or brackish water in estuaries (Ellison et al., 1999). Mangrove forests have extensive canopies and aerial and submerged root systems. Associated with these root systems is a diverse assemblage of macroalgae, microalgae and microorganisms (Alongi, 2012). Mangrove forests provide breeding sites, nursery grounds and habitat for fish, sharks, sea turtles, sea horses, shrimp, mangrove crabs, blue crabs, tree crabs, horseshoe crabs, lobsters, snails, starfish, oysters, mussels, barnacles, sea anemones, sponges, bryozoans, polychaete worms and jellyfish. Fish species include mudskippers, tarpon, sheepshead, jacks, drum, grunts, sea trout, snappers and mullet. They also support birds, snakes, lizards, mammals and alligators.
How much Blue Carbon do Mangrove Forests sequester?
Globally, mangrove forests sequester between 4 to 20 Pg of blue carbon (i.e., 4 to 20 billion tons of carbon). Donato et al. (2011) estimated mangrove forests sequester about 1023 Mg of blue carbon per hectare with about 159 Mg of this blue carbon being located above ground. Donato et al. also found that mangrove forests store between 71-98% of their blue carbon below ground in estuaries and 49-90% below ground in oceanic areas. Alongi (2012) found that 75-95% of below ground mangrove forest carbon was located in dead, rather than, live roots. Alongi found that mangrove forests utilize these dead roots for nutrients and also as pathways to grow new roots. The ability of mangrove forests to sequester vast amounts of blue carbon below ground makes them an important ecosystem that can be utilized to help mitigate Climate Change and Global Warming.
Threats to Mangrove Forests
Mangrove forests are one of the most threatened tropical ecosystems (Valiela et al., 2001). They are disappearing at a rate of 1 to 3% per year (Giri et al., 2011/Duarte et al., 2008/Alongi, 2012). Globally, over half of the mangrove forests have been destroyed due to urban development, aquaculture, mining and overexploitation for timber, fish, crustaceans and shellfish (Giri et al., 2011/Duke et al., 2007/Alongi, D.M., 2002). In addition, a major percentage of existing mangrove forests are degraded (Giri et al., 2011). Some scientists believe that they may completely disappear within the next 100 years if measures are not taken to protect and restore them (Duke et al., 2007). Mangrove forests not only sequester blue carbon but also protect coastlines from hurricanes, storm surge and erosion; serve as nurseries for commercially important fish species; and provide renewable wood products. The destruction of mangrove forests results in the loss of the above environmental services and the release of greenhouse gases from disturbed mangrove forest soils. Impacted mangrove forests currently release between 0.02 to 0.12 Pg of blue carbon per year (Donato et al., 2011).
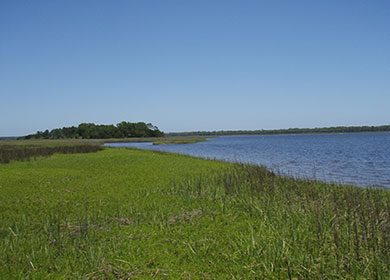
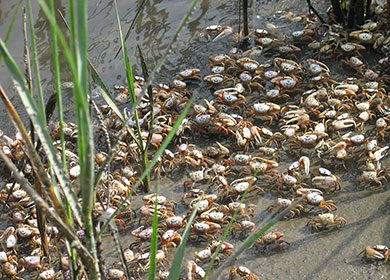
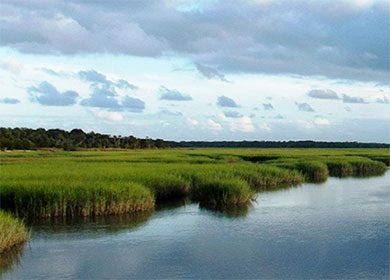
Salt Marshes
Salt marshes are Blue Carbon coastal intertidal wetlands with herbaceous, salt-tolerant plants such as grasses in the genus Spartina (Sporobolus). Salt marshes are located in low wave energy coastal areas where the waters of freshwater rivers and the ocean meet (i.e., estuaries). They are often protected from waves by barrier islands and are located in colder temperate regions where mangroves are restricted by freezing temperatures. Salt marshes cover approximately 22,000 to 400,000 km² world-wide (McLeod et al., 2011) making them one of the largest Blue Carbon ecosystems on the planet.
Similar to seagrass meadows and mangrove forests, salt marshes are very important nurseries for numerous fish and crustaceans. The stems of marsh grasses like Spartina alterniflora or smooth cordgrass form dense stands that provide excellent cover or protection for juvenile fish and crustaceans that are hiding from predators. In a review of 32 salt marsh studies, Minello et al. (2003) found that the juveniles of 28 fish species, 2 crab species and 5 shrimp species utilize salt marshes as nurseries. Important commercial and recreational fish and crustacean species included white and brown shrimp, blue crabs, Gulf menhaden, mullet, flounder, sheepshead, mojarra, pinfish, whiting, redfish, spotted seatrout, ladyfish and Atlantic croaker.
In a literature review to document the use of salt marshes by megafauna (large animals), Gaskins et al. (2020) found that 34 megafaunal species around the world use salt marshes and help shape these communities through their actions. In southeastern U.S. saltmarshes, white-tailed deer, Florida panthers, wild boar, wild horses, black bear, American crocodile, bottlenose dolphins, bull sharks, American alligators, and West Indian manatees use these ecosystems. In west coast U.S. salt marshes, mule deer, sea lions, sea otters and harbor seals utilize the marshes. Ostriches, African buffalo, Nile crocodile, hippopotamuses, zebra, lions, springbok and warthogs have been found in African salt marshes.
How much carbon dioxide or Blue Carbon can Salt Marshes sequester?
Globally, salt marshes sequester between 4.8 to 87.2 TgC per year (McLeod et al., 2011). Carbon burial rates for salt marshes have been estimated in the range of 18 to 1713 grams of carbon per m² per year (Chmura, 2013). Based on the large ranges in the estimates above, research needs to be done to develop better estimates and a deeper understanding of the importance of salt marshes in storing Blue Carbon and mitigating Global Warming. Based on the high estimates of the global area of salt marshes, burial rates of carbon and their storages of Blue Carbon, salt marshes may be the most important Blue Carbon ecosystem of the planet.
What are the threats to Salt Marshes?
Due to their location, accessibility, elevation and resources, salt marshes have been used and impacted by human societies for thousands of years. Impacts have occurred due to practices including harvesting, farming, grazing, draining, dredging, filling, developing, salt production, and the dumping of sewage and trash (Laffoley and Grimsditch, 2009/Gedan et al., 2009). Salt marshes are currently disappearing at a rate of 1 to 2% per year (Duarte et al., 2008).
Sea level rise due to Global Warming is a major threat to salt marsh ecosystems. Coastal researchers are currently trying to determine what will happen to salt marsh carbon storages once they are inundated by rising seas. With existing coastal development and land uses, large areas of salt marshes around the world may not be able to migrate inland and might disappear due to sea-level rise. With their disappearance will be the loss of their ecosystem service of sequestering vast amounts of Blue Carbon.
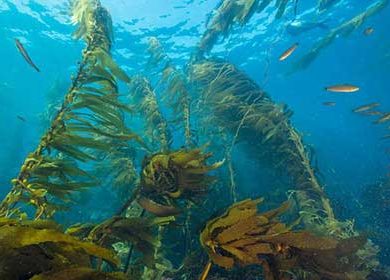
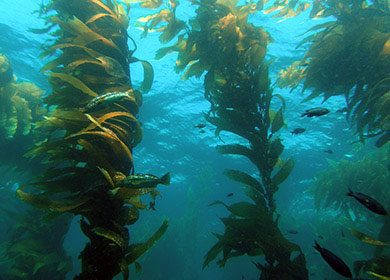
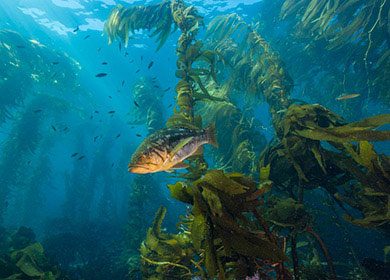
Kelp Forests and other Macroalgae Communities
Macroalgae are blue carbon ecosystems that consist of tropical and temperate species that include red, brown, green and blue-green algae. Globally, macroalgae sequesters approximately 173 teragrams of blue carbon per year (or about 381 billion pounds of carbon per year/ Krause-Jensen and Duarte, 2016). Blue carbon sequestered in the tissues of macroalgae is often carried by currents away from their natural shallow water habitats and deposited and stored in sediments of the continental shelf and deep ocean. Eighty two percent of the primary production of kelp forests, for example, which have gas-filled bladders that make kelp float, are deposited away from where the forests grow (Krumhansl and Scheibling, 2012).
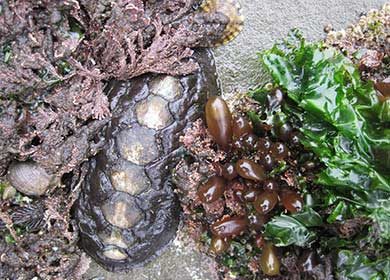
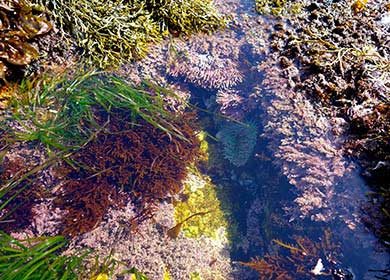
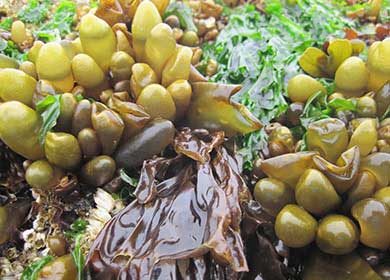
References
- Alongi, D.M., 2002. Present state and future of the world’s mangrove forests. Environmental conservation, 29(3), pp.331-349.
- Alongi, D.M., 2012. Carbon sequestration in mangrove forests. Carbon management, 3(3), pp.313-322.
- Chmura, G.L., 2013. What do we need to assess the sustainability of the tidal salt marsh carbon sink? Ocean & Coastal Management, 83, pp.25-31.
- Coverdale, T.C., Brisson, C.P., Young, E.W., Yin, S.F., Donnelly, J.P. and Bertness, M.D., 2014. Indirect human impacts reverse centuries of carbon sequestration and salt marsh accretion. PLoS One, 9(3), p.e93296.
- Donato, D.C., Kauffman, J.B., Murdiyarso, D., Kurnianto, S., Stidham, M. and Kanninen, M., 2011. Mangroves among the most carbon-rich forests in the tropics. Nature geoscience, 4(5), pp.293-297.
- Duarte, C.M., 2002. The future of seagrass meadows. Environmental conservation, 29(2), pp.192-206.
- Duarte, C.M., Dennison, W.C., Orth, R.J. and Carruthers, T.J., 2008. The charisma of coastal ecosystems: addressing the imbalance. Estuaries and coasts, 31(2), pp.233-238.
- Duarte, C.M., Losada, I.J., Hendriks, I.E., Mazarrasa, I. and Marbà, N., 2013. The role of coastal plant communities for climate change mitigation and adaptation. Nature Climate Change, 3(11), pp.961-968.
- Duarte, C.M., Marbà, N., Gacia, E., Fourqurean, J.W., Beggins, J., Barrón, C. and Apostolaki, E.T., 2010. Seagrass community metabolism: Assessing the carbon sink capacity of seagrass meadows. Global Biogeochemical Cycles, 24(4).
- Duke, N.C., Meynecke, J.O., Dittmann, S., Ellison, A.M., Anger, K., Berger, U., Cannicci, S., Diele, K., Ewel, K.C., Field, C.D. and Koedam, N., 2007. A world without mangroves?. Science, 317(5834), pp.41-42.
- Ellison, A.M., Farnsworth, E.J. and Merkt, R.E., 1999. Origins of mangrove ecosystems and the mangrove biodiversity anomaly. Global Ecology and Biogeography, 8(2), pp.95-115.
- Fourqurean, J.W., Duarte, C.M., Kennedy, H., Marbà, N., Holmer, M., Mateo, M.A., Apostolaki, E.T., Kendrick, G.A., Krause-Jensen, D., McGlathery, K.J. and Serrano, O., 2012. Seagrass ecosystems as a globally significant carbon stock. Nature geoscience, 5(7), pp.505-509.
- Gaskins, L.C., Paxton, A.B. and Silliman, B.R., 2020. Megafauna in salt marshes. Frontiers in Marine Science, 7, p.975.
- Gedan, K.B., Silliman, B.R. and Bertness, M.D., 2009. Centuries of human-driven change in salt marsh ecosystems.
- Giri, C., Ochieng, E., Tieszen, L.L., Zhu, Z., Singh, A., Loveland, T., Masek, J. and Duke, N., 2011. Status and distribution of mangrove forests of the world using earth observation satellite data. Global Ecology and Biogeography, 20(1), pp.154-159.
- Green, E.P., Short, F.T. and Frederick, T., 2003. World atlas of seagrasses. Univ of California Press.
- Krause-Jensen, D. and Duarte, C.M., 2016. Substantial role of macroalgae in marine carbon sequestration. Nature Geoscience, 9(10), pp.737-742.
- Krumhansl, K.A. and Scheibling, R.E., 2012. Production and fate of kelp detritus. Marine Ecology Progress Series, 467, pp.281-302.
- Laffoley, D. and Grimsditch, G.D. eds., 2009. The management of natural coastal carbon sinks. IUCN.
- Lo Iacono, C., Mateo, M.A., Gracia, E., Guasch, L., Carbonell, R., Serrano, L., Serrano, O. and Danobeitia, J., 2008. Very high‐resolution seismo‐acoustic imaging of seagrass meadows (Mediterranean Sea): Implications for carbon sink estimates. Geophysical Research Letters, 35(18).
- Lovelock, C.E. and Duarte, C.M., 2019. Dimensions of Blue Carbon and emerging perspectives. Biology letters, 15(3).
- Minello, T.J., Able, K.W., Weinstein, M.P. and Hays, C.G., 2003. Salt marshes as nurseries for nekton: testing hypotheses on density, growth and survival through meta-analysis. Marine Ecology Progress Series, 246, pp.39-59.
- Nellemann, C., Corcoran, E., Duarte, C.M., De Young, C., Fonseca, L.E. and Grimsdith, G., 2010. Blue carbon: the role of healthy oceans in binding carbon.
Short, F.T. and Wyllie-Echeverria, S., 1996. Natural and human-induced disturbance of seagrasses. Environmental conservation, 23(1), pp.17-27.
- Valiela, I., Bowen, J.L. and York, J.K., 2001. Mangrove Forests: One of the World’s Threatened Major Tropical Environments: At least 35% of the area of mangrove forests has been lost in the past two decades, losses that exceed those for tropical rain forests and coral reefs, two other well-known threatened environments. Bioscience, 51(10), pp.807-815.